BIOSYNTHESIS
OF EUMELANIN AND PHEOMELANIN
Richard Cryberg
Introduction
Biosynthesis of eumelanins is widespread in the plant and animal
kingdoms. In plants commonly recognized colorations that are due
to eumelanin like substances are such things as browning of apples or
bananas or blackening fresh cuts on potatoes. Much of the brown
and black coloration seen in various fungi is due to eumelanin like
substances. However, not all dark plant-produced pigments
are melanins. Many are due to various other polymerized quinone
structures such as napthaquinones or anthraquinones. These are
often formed as a result of the action of oxygen and tyrosinase, the
key enzyme at the start of melanin synthesis, acting on the appropriate
non-protein substrate. Thus from an evolutionary standpoint
formation of these types of pigment preceded the development of the
melanin pathways 1(ff1).
In the mammals the main coloring agents are melanins in addition to
pinks from hemoglobin in blood close to the surface. Fish,
reptiles and birds have two other common color forming mechanisms,
lipochromes and structural colors. Lipochrome simply means
fat-soluble. Brilliant reds and yellows formed from carotene or
its derivatives are commonly deposited in birds fat, feathers and skin
or scales. The vivid yellows and reds such as seen in canaries
for yellow or a cardinal for red are examples. The red on a
pigeon’s leg is lipochrome based2. More muted yellows
and reds
seen in mammals or birds are often due to pheomelanins. The
so-called structural colors result from diffraction and refraction of
light by the microstructure of feathers or scales to create a variety
of colors ranging from blues to iridescence. A great many birds
show some effects due to structural colors on at least part of the
feathers. In general any blue seen in animal life is a structural
color. In both mammals and birds there are also some other minor
pigment based colors that in some cases are very important. As it
is not obvious that these exist in pigeons I am going to skip them.
As a point of interest, the black ink from squid is among the most
concentrated suspensions of melanin available. This ink is
largely uncontaminated with proteins and other cellular products and
therefore has been of great value in learning about the chemical makeup
of melanin.
A few words about how this is organized might help the reader.
The material presented in the main body is out of the published
scientific literature and is well documented. Most of it has been
learned from species other then pigeons. As biological processes
are well preserved across species what we learn about pigment synthesis
in animals other then pigeons is in general directly applicable to
pigeons. I have added a number of footnotes which contain
unpublished observations I have made as well as what seem
straightforward interpretations as applied to pigeons. Without
doubt some of my interpretations will prove modestly incorrect in the
details as science moves forward.
Also I need to be very clear that nothing I say in this paper has
anything at all to do with formation of the white areas of any of the
various pied varieties. The white areas in pied are the result of
things like differential and selective migration of melanocytes in the
embryo or with programmed cell death of melanocytes and not with
turning pigment synthesis on in some regions of the birds body and off
in others. In pied variants the feathers in an area are either
white or colored. The colored areas of pied are governed by the
rules given herein including those where the colored feathers show both
color and white.
Plant Kingdom
Melanins
The chemistry of formation of eumelanins in the plant kingdom seems to
have been fairly well worked out 3. There seem to be
only three
essential ingredients. They are the enzyme tyrosinase, the amino
acid tyrosine and oxygen. Pheomelanins are unknown in the plant
kingdom. Tyrosine is oxidized to dopa that is then oxidized
again to dopaquinone. It is clear that both the enzyme tyrosinase
and oxygen are essential for the second reaction, conversion of dopa to
dopaquinone. The exact chemistry of the first reaction is less
clear. During in vitro studies mixing tyrosinase, tyrosine and
oxygen only leads to formation of dopa after a significant induction
period. A considerable amount of effort has been expended to
understand the basis of this induction period 4. It
seems to
mainly be a result of needing time to build up a small amount of
dopaquinone. Adding preformed dopaquinone or various metal ions
to the reaction mix can considerably shorten the induction
period. This preformed dopaquinone then acts as the true oxidizer
in conversion of tyrosine to dopa. It is unclear what role the
metals play although effective metals have two readily available
oxidation states and likely simply act as co-oxidants together with the
tyrosinase. It is not clear if metals play a role in biological
systems.
Dopaquinone, together with atmospheric oxygen is capable of slowly
forming a variety of brown and black pigments in in-vitro
experiments. The rapidity of color formation and the color formed
varies with conditions such as pH or presence of various free metal
ions over and above the bound copper found in tyrosinase. This
plant based or in vitro produced pigment does not have the stability
nor molecular complexity of animal produced melanins. They are
much lower in molecular weights also.
Amimal Kingdom
Melanins
Tyrosinase
In animals there is a regulatory scheme that controls the production of
tyrosinase as shown in Chart 1 5. A key step in this
chart is
that MC1R modulates the activity of the tyrosinase produced. This
modulation is caused by phosphorylation of the tyrosinase produced by
the gene and increases the enzymatic activity. Different
activities of tyrosinase lead to very different phenotypes as low
activities cause the chemistry to proceed mainly down the pheomelanin
path while intermediate or high activities force the chemistry down the
eumelanin path 6. Dominant MC1R mutants are not at all
uncommon which
lock the receptor site in a full on state 7, 8,9,10.
These full
on states are called constitutive mutations. With these mutations
the MC1R site is in a full on state even without the help of
melanocortin stimulating hormone. In these full on states
production of eumelanin is very high leading to black or near black
phenotypes. There are also some other kinease enzymes involved in
the overall modulation scheme. Their exact role is not yet
understood in detail. Nonetheless, it is clear that the things
that happen before actual pigment synthesis even starts govern much of
the final color produced.
Chart
1. Formation of Tyrosinase
Eumelanin Formation
Chart 2 summarizes the well-proven chemistry of eumelanin formation
11,12. Many of the steps have been proven by
techniques such as
adding model compounds to tissue samples where the model compound would
be expected to only be able to move one or two steps down the synthesis
pathway before stopping due to not fitting the needs of further
steps. All intermediates shown have been either isolated or
proven to exist as transient species by such instrumental techniques as
nuclear magnetic resonance or mass spectral examination of reaction
mixtures during the course of the reaction.
Clearly all the details of what happens in the processes in this chart
are not yet known. For instance there must be enzymes that
release free tyrosine as raw material for melanin synthesis
involved. Tyrosine deficient diets are known to reduce pigment in
animals. Other mutants may well produce products that promote or
antagonize each step in this early part of the pathway resulting in
increased or decreased pigment levels. The point is that by
considering the steps shown and such promotion or antagonism affects a
great many of the known color mutants can be explained even before
pigment synthesis starts. This illustrates why much of the future
progress in understanding exactly what causes different mutant affects
is going to be discovered in lab studies and not in the breeding
loft. As breeders our main job is to find and characterize new
mutants these days. That cannot be done in the lab, at least not
just yet.
Chart
2. Biochemical Synthesis of Emuelanin
Tyrosinase seems essential only for the first two chemical steps that
result in dopaquinone. While tyrosinase is not essential it may
promote some of the subsequent reactions in vivo. The wild type
gene responsible for production of tyrosinase is universally recognized
as the locus responsible for albinism when the gene is defective.
Tyrosinase becomes more complex in higher life forms, but essentially
always consists of a fairly large protein which complexes copper.
In plants and fungi only one copper is present per tyrosinase. In
vertebrates tyrosinase is a considerably larger molecule and complexes
four coppers. It is this copper which is the actual oxidant in
conversion of dopa to dopaquinone. The oxygen required is simply
to reoxidize the reduced copper. Any genetic defect which makes
the enzyme incapable of binding copper will render the enzyme 100%
ineffective with the end result being the organism is melanin free and
a pure albino. But, as often happens in biology we know of
examples of near albinos where the enzyme apparently is rendered almost
inactive but not quite 100%. In these cases the copper binding
sites are intact but the enzyme does not have the other structural
characteristics needed to be an effective catalyst. In humans we
know today of over 60 alleles of this gene that lead to albinism 13
(ff2).
It seems sure that some genetic influence impacts some steps in this
sequence. However at this date no genes have been proven to
influence the chemistry. The eumelanin pathway leads to brown and
black products and in very rare cases to red products. Dopachrome
itself is red and has been isolated as an end product from the sea worm
Halla parthenopea 14. Also in hair some of the very
reddest hair
examples lead to degradation products consistent with eumelanin.
Elemental analysis of this red pigment is inconsistent with pheomelanin
15. Little seems to be definitely known about what
exactly causes
eumelanin to range in color from red on rare occasions to the normal
browns and blacks. The reds seem to involve some oxidative
degradation of the 5,6 dihydroxyindole units resulting in bleaching
much as happens in hair treated with hydrogen peroxide. The
difference between black and brown seems to be in part due to the size
and shapes of the pigment particles as well as total pigment
concentration. Size and shape can affect the way light is
reflected and refracted causing different perceived colors. A
dark brown pigment will appear black when at high concentrations.
It is also widely accepted that melanins can consist of heteropolymers
made of monomers from both eumelanin and pheomelanin 16.
Such
mixed polymer molecules could appear brown in color. However, it
is also clear that some well explored species of domestic birds are
known that produce both browns and blacks but at least to date have
never been known to produce reds via pheomelanins. Canaries and
geese are good examples. So such mixed polymers are not essential
to production of brown pigments. It is clear, without doubt, that
whatever causes phenotypic color to shift between black and brown
happens during or after formation of the polymeric products.
There is absolutely nothing in the small molecule chemistry that
impacts this color shift.
Pheomelanin Formation
At the dopaquinone step the synthesis pathway forks. Chart 3
shows the chemistry after this fork. The amino acid cysteine combines
with dopaquinone followed by chemistry of this adduct, eventually
producing the well-recognized red pheomelanin pigments. Which
fork the chemistry follows is largely a matter of tyrosinase
activity. At low activity of tyrosinase the fork to pheomelanin
is followed 17,18 while at high activity the fork to
eumelanin is
followed. As the tyrosinase activity is modulated by MC1R, as
shown in chart 1, MC1R turns out to be the primary determinant in red
pheomelanin versus brown/black eumelanin pigment
production. There are many known recessive alleles of the
MC1R gene that in effect break the function of the site resulting in
red hair or feather color. In mice four recessive alleles are
well-characterized 19. Recessives are also known in
many other
birds and animals. All lead to red/yellow phenotype feathers or
hair. This modulation of tyrosinase activity can also be turned
on and off by organisms. Many animals are known where part of the
hair is colored with pheomelanin and another part with eumelanin.
We clearly see this effect in many of the pigeon mutants where both
colors are visible on different areas of the same feather.
Chart
3. Biochemical Synthesis of Phoemelanin
As is the case in eumelanin little is known about the actual pigment
formation process as soon as the small molecule sequence ends.
Again much of the small molecule chemistry after formation of the
dopaquinone-cysteine adduct is formed seems to be close to spontaneous
other then needing peroxidases at a couple of stages. Just before
the final polymerization trichromes are formed which are poorly
characterized mixes of compounds that seem to be dimers and
trimers. These materials are red in color and have been isolated
in small amounts from biological organisms as seemingly final
products. It is believed that trichromes are generally involved
in the subsequent polymerization to form pheomelanin. There are
no known genes involved in the chemical steps subsequent to formation
of dopaquinone. This will ultimately prove to be untrue as
undoubtedly genes are involved that control pigment polymerization,
particle size and shape of pigment produced and pigment transport
processes in both the eumelanin and pheomelanin pathways. But
most such genes may well prove to have little impact on phenotypic
appearance (ff3).
In some species of birds the ability to make pheomelanin seems to be
absent. Perhaps this is because they do not have sufficient free
cysteine present to allow production of the cysteine adduct with
dopaquinone. In such cases broken versions when homozygous simply
result in a white bird. An example would be snow geese 20.
Interestingly this is the only example of a co dominant MC1R allele
that I have run into in my searches. It would be reasonable to
expect that some versions of melanocyte stimulating hormone might have
intermediate activity and thus lead to co dominance but in the snow
goose case the specific defect was identified in the MC1R gene itself.
As many animals are known to have upwards of 50 different genetic loci
that impact the final color and pattern of pigment deposition it is
obvious that the above chemical steps and known associated genes are
only a fragment of the whole color forming process. However it
needs to be pointed out that many of the pigment deposition patterns
found in feathers have been mathematically modeled and found to follow
fairly simple differential equations 21. This paper
shows
pictures of computer-generated solutions to the equations used while
substituting various values into the constants. Clearly the
simulations generated essentially all the color distribution patterns I
have ever seen on real feathers as well as the patterns I have seen
under microscopic examination of feather parts. This illustrates
that great variation in phenotype can be achieved by very simple means
with surprisingly simple genetic control. The math used is much
the same as the math that has been used for many years to model
oscillation in electronic as well as chemical systems. All that is
required to see real life feathers showing exactly the same affects is
a relatively simple feedback mechanism in the chemistry of the pigment
forming steps. For example a gene that produced a product
that both increased the production of tyrosine while also dropping in
the production of this gene product in proportion to any one of the
chemical products of pigment synthesis would fill the need. What
the above paper shows is by adjusting the intensity of feedback
essentially any pattern of pigment deposition can be achieved.
Such simple processes can easily account for bars and checks for
instance. Or the many beautiful black stripes you see on the blue
area of a pigeon feather at magnifications of only 50 or 100X.
We also know of bacterial studies that show that simple random noise
can have phenotypic outcomes 22,23. Random noise in
the pigment
production process could easily explain such genetic effects as seen in
the grizzle or almond series of alleles where pigment production is
turned on and off and even back and forth between two different
pigments. Just as stable oscillations can be governed by
relatively simple feedback systems random noise can be generated by the
very same systems. In some cases all that is needed is to choose
different constants for the same mathematical equations (ff4).
Acknowledgements
This paper would not have been possible without the help of several
others who deserve recognition. My wife, Dawn, did a significant
portion of the internet searches and also cajoled our local library to
get copies of several papers and also books on interlibrary
loans. Much of this information was critical in writing the paper.
Dr. Daniel Smith read and gave very helpful suggestions during the
writing process. Most of what is presented on the mode of MC1R
action comes directly from his study of the technical literature.
Without his help this critical part of the pigment forming process
would not have been covered in anything close to an adequate fashion.
David Rinehart also deserves recognition. David was responsible
for prodding me into starting down this study path and has cajoled and
tormented me regularly for the past six months to get me to
continue. He is also the person who made me aware that genetic
noise was a factor that needed to be considered when we talk about
genetic mechanisms. His very challenging questions have kept me
thinking through the whole process of trying to learn about the known
technology of pigment formation. It was at his insistence that I
got out my optical microscope and learned to see pigment particles and
take pictures of what I saw.
I also need to recognize those who laid the groundwork in pigeon
genetics for all the fine background information and thought provoking
questions that they raised. In particular Hollander, Sell and
Quinn wrote books and papers containing actual data that are relevant
and timely even if dated in some cases. We need more actual
published data to further our understanding.
Footnotes
ff1. Enough is know today about melanin chemistry that we can
make a fair reconstruction of the total evolutionary pathway that has
occurred. At some early time our single cell ancestors had a
problem with UV light. At that early point in earth’s history
there was no oxygen in the atmosphere and thus no ozone layer to shield
the surface from UV. Thus while all life was then confined to the
oceans the UV meant that organisms could not even venture too close to
the surface. Some primitive organism accidentally made a gene
that produced a protein that bound copper and had enough oxidation
activity to convert naphthalene adducts to napthaquinones.
Quinones in general are black and excellent UV absorbers, thus the
organism derived some reproduction advantage and passed on its new
gene. When we look at the tyrosinase in today’s primitive
organisms we find that they are small proteins and only bind one copper
per molecule.
At some point fairly early on the organism had another genetic
accident. This accident produced enough free tyrosine in the cell
to allow interaction with the already existing tyrosinase and oxidation
of the tyrosine to dopaquinone. The dopaquinone spontaneously
underwent the needed reactions, aided by peroxidases where oxidation
was required, to produce the first primitive melanin like
products. Again we find exactly this mechanism at work in some
fungi and plants today.
With time the organism had more molecular accidents that resulted in
duplication of the tyrosinase gene. Perhaps a deletion or
duplication in a noncoding region near the gene prevented exact lineup
during sexual cell division and the nonaligned cross over resulted in
one cell winding up with two primitive tyrosinase genes end to
end. The new gene had two copper binding sites and was a more
efficient enzyme then the original version. In fact, in today’s
higher animals the tyrosinase enzyme has doubled again and binds four
coppers and is several times the size of the tyrosinase found in
today’s primitive organisms.
While the evolution of the tyrosinase enzyme was happening the rest of
the cellular processes we find in modern animals was also evolving such
that eventually pigment was only produced in special cells at in areas
where it was actually needed. Further the polymerization of the
small molecules was better controlled to produce stable pigment in of
the best shape and particle size to provide UV protection.
Well down the evolutionary pathway, well after multicell animals had
evolved, an organism had a genetic accident that resulted in enough
free cysteine being present in the same cells making tyrosinase that
pheomelanins could be produced. And today’s animals live with the
benefits of all these genetic accidents.
So when the anti evolution crowd say complex processes are too complex
to have evolved by accident they simply show a lack of understanding of
how simple accidents far back in time can have been modified and
improved in many small accidental steps and result in the complex
biochemical processes we see today. And it has only been in the
last few years that sciences like biochemistry, biology, chemistry,
engineering and genetics have been forced to start cooperative efforts
that digging out the details of how such complex processes happened in
small steps could be identified.
ff2. If having 60 alleles at one gene locus seems excessive I
suggest it is far past time for the pigeon community to get used to the
idea of multiple alleles. When examined at the molecular level
multiple alleles are proving the norm rather then the exception.
For the human disease cystic fibrosis we now know of over 1000 alleles
of the CF gene. We are adding new alleles at a rate of over ten
per month these days. There is even a commercial company that has
a screen in place to determine if an individual has one of more of over
1000 known defects in his genome. The present cost is roughly $3
per defect screened for by this company.
ff3. Joe Quinn with remarkable insight anticipated what we would
learn about the chemistry of pigment formation when he wrote “The
Pigeon Breeders Notebook.” In that book he suggested considering
all the bronzing genes as possible alleles of each other suggesting
that he believed that on a chemical level the same chemical pathway
caused all bronzes 24. Well, Quinn came very close to
getting it
right. He just stopped a little too soon. In fact we know
today that while all the bronzes are not alleles (but so did Quinn)
exactly the same chemistry produces the pigment in every single one of
them. Further it is obvious that a variety of other genes, such
as ash red, indigo, recessive red and recessive opal, also exert their
influence at this same chemical step. In fact, we now know that
all pheomelanin pigment produced results from some action that causes
dopaquinone to react with cysteine rather then simply ring close to an
indole. And we also know without doubt that many of the reds are
really pheomelanin and not some unusual eumelanin thanks to the
quantitative analyses of feather pigments published by Sell et al 25.
Now, many of these bronzed colors also are perfectly capable of
making eumelanin as well as pheomelanin. For example I have
microscopically examined the tail feathers of a hen ash red bird and
clearly shown the presence of black eumelanin. It has made little
sense for quite a few years to think that ash red and brown were
actually alleles rather then simply closely linked genes.
Understanding the chemical pathways that lead to pheomelanin vs
eumelanin and understanding that the choice between black and brown
eumelanin takes place at a very different point in the chemical pathway
is simply another proof that ash red is not a brown allele. As
soon as you realize they are not alleles it is easy to understand how
an ash red hen can make black eumelanin.
We have been sitting on phenotypic clues for years that hinted that
many of the bronzing effects were caused by the same or very similar
mechanisms. Consider how much some homozygous recessive opals and
homozygous indigos look like ash red for example. I think it is
very likely that the feedback system that turns red pigment production
on and nearly turns black pigment production off acts on exactly the
same one or two steps in the synthesis pathway for all the bronzing
genes. In fact it would be far more unlikely for this to not be
true. While we know that it takes low activities of tyrosinase to
produce red pigment this is not the whole story. What we do not
know is what this low activity must be relative to. For instance
is it a low activity of tyrosinase relative to tyrosine or relative to
dopa that is important? Or perhaps relative to dopaquinone or
tyrosine? And are there other enzymes that also get in the
act? Until we know answers to these kinds of questions we are not
going to know the whole story.
We are missing important genes that we should be seeing in
pigeons. For instance either recessive red or spread in pigeons
is the same as the MC1R gene. But it cannot be both as recessive
red and spread are not even on the same chromosome. In other
species such as mice where large numbers of animals have been raised it
is common to have both recessive red and a gene analogous to our spread
gene as alleles. So in pigeons we are either missing the dominant
black phenotype that is an allele of recessive red or we are missing
the recessive red phenotype that is an allele of spread. It seems
more likely to me that when the gene sequencer guys have the answer we
will find that our recessive red is equal to MC1R. My reason for
leaning this way is easy. In other species there are generally
more alleles for the recessive red phenotype then for the spread
phenotype. Yet in pigeons we know of only two alleles for
recessive red. And ember is clearly less broken then
recessive red itself. But we have hints of a whole variety of
alleles at the spread locus. Some of the later do not even darken
the albescent strip. And others need all kinds of help to produce
a decent solid black. Some are able to turn a blue bar into a
very nice black with no other darkeners. There are a lot more
ways in general to break a gene then lock it full on. I think we
simply have too many spread alleles to make it the best choice for
MC1R. It will be great when we know for sure. The
possibility exists that we do have the constitutive gene for MC1R and
simply have not recognized it. We know that both ember and
recessive red do not cover the albescent strip on the tail feathers
without help form other darkening genes. We also know of apparent
spread genes that leave this strip unchanged from wild type. No
one, as far as I know, has ever taken one of these poor spread
phenotypes and checked to see if it was actually a spread allele or if
it might instead be an allele of recessive red. There is no real
reason at this point to rush to the breeding loft and make such tests
as we will probably know from lab studies shortly if recessive red is
in fact the MC1R locus and at that point it will be far easier to check
various spread variants in the lab and see if any are also at the MC1R
locus.
The color brown in pigeons remains a bit of a puzzle. As I have
pointed out above brown coloration is not well understood. Sell,
et al showed clearly that a brown bar pigeon had less eumelanin and
more pheomelanin in the bar areas then the blue counterpart. In
the smooth spread areas this same bird had considerably less eumelanin
and almost no pheomelanin compared to wild type. In spread brown
both eumelanin and pheomelanin were considerably lower then in spread
black. Brown is well known to bleach easily when exposed to
sunlight. While the lower concentrations of eumelanin could tend
to look brown rather then black the bleaching is a strong hint that the
pigment in browns is already partially degraded upon deposition in the
feather. Work that shows that eumelanin based reds are actually
the result of oxidative damage to the eumelanin would fit with our
brown pigeons simply being due to lightly oxidized eumelanin.
Once some oxidation has happened it is generally easier chemically to
get further oxidation. Pigments exposed to sunlight are going to
absorb UV and get kicked into either singlet or triplet states that can
easily combine with atmospheric oxygen. Such damage would be
expected to show up to naked eye exam faster on browns then any other
color due to the relatively low total pigment concentrations.
Just to make life interesting there are also some bird species known
that are brown because of porphyrin based pigments 26.
These
pigments are reported to be the main coloring agents in the feathers of
bustards, owls and goatsuckers. I know of no evidence that these
pigments are either light sensitive or in pigeons.
ff4. I do not want to get bogged down in a lot of math. In
the first place I would have to go back and relearn all kinds of stuff
I have not thought about for over 40 years except in nightmares.
For anyone who is interested a good EE text on the math of electrical
feedback systems would be a good place to start. Then follow up
with one of the recent books on chaos theory. Between the two it
should be rapidly obvious that simple linear and nonlinear feedback
systems that tied into the front end of pigment synthesis can easily
generate any of the pigment patterns we see, either naked eye or
microscopically. When I say pigment patterns I am referring to
bars, checks and various bicolor types. I am not talking about
pied types. These patterns include the effects seen in all the
grizzles and almonds. It is mainly a matter of picking the
correct constants to feed into the equations. By including the
effect of low activities of tyrosinase leading mainly to pheomelanin
and high leading to eumelanin you could easily understand why grizzles
often show bronzing, particularly in the transition between eumelanin
areas and white areas. All you need to do is drop the tyrosinase
concentration a bit slowly while in the eumelanin production mode and
pigment production will switch to bronze and then white at a very low
activity of tyrosinase. No gene other then the grizzle gene is
needed to explain such bronzing. Exactly the same sequence would
explain what we see in almond.
References:
1. Giuseppe Prota, Melanins and Melanogenesis, Academic Press Inc
(London) Ltd, 1992, pp. 10-11.
2. W. F. Hollander, Origins and Excursions in Pigeon Genetics, pp. 31,
141.
3. Giuseppe Prota, Melanins and Melanogenesis, Academic Press Inc
(London) Ltd, 1992, pp. 1-7.
4. Giuseppe Prota, Melanins and Melanogenesis, Academic Press Inc
(London) Ltd, 1992, pp. 42-57.
5. Professor Daniel A. Smith, Chemistry Department, Goshen College,
private communication.
6. S. Kerie, J. Lind, K. Schutz, P. Jensen and L. Andersson,
Melanocortin 1-receptor (MC1R) mutations are associated with plumage
colour in chicken, Animal Genetics, 34, pp. 241, August
2003. Also web
address:
http://www.blackwell-synergy.com/doi/abs/10.1046/j.1365-2052.2003.00991.x?journalCode=age
7. E.Theron, K. Hawkins, E. Bermingham, RE. Ricklefs, NI. Mundy, The
molecular basis of an avian plumage polymorphism in the wild: a
Melanocortin-1-receptor point mutations is perfectly associated with
the melanic plumage morph of the bananaquit, Coereba flaveola. Web
address:
http://www.ncbi.nlm.nih.gov/entrez/query.fcgi?cmd=Retrieve&db=PubMed&list_uids=11369199&dopt=Abstract
8. Maria K. Ling, Malin C. Lagerstrom, Robert Fredriksson, Ronald
Okimoto, Nicholas I. Mundy, Sakea Takeuchi and Helgi B. Schioth,
Association of feather colour with constitutively active melanocortin 1
receptors in chicken, Eur. J. Biochem. 270, pp.
1441-1449(2003). Web address:
http://content.febsjournal.org/cgi/content/full/270/7/1441
9. Dongsi Lu, Dag Inge Vage and Roger D. Cone, A Ligand-Mimetic Model
for Constitutive Activation of the Melanocortin-1 Receptor, Molecular
Endocrinology 12(4): pp. 592-604, web address:
http://mend.endojournals.org/cgi/content/full/12/4/592
10. S.M. Doucet, M.D. Shawkey, M. K. Rathburn, H. L. Mays Jr, and R.
Montgomerie, Concordant evolution or plumage colour and feather
microstructure and a Melanocortin receptor gene between mainland and
island populations of a fairy-wren, Proc. R. Soc. Lond. B, pp. 1-8,
2004, web address:
http://web6.duc.auburn.edu/~maysher/fairy%20wren%20color%20paper.pdf
11. Aaron Bunsen Lerner and Thomas B Fitzpatrick, Biochemistry of
Melanin Formation, Physio. Rev., 30: pp. 91-126(1950)
12. Giuseppe Prota, Melanins and Melanogenesis, Academic Press Inc
(London) Ltd, 1992, pp. 6.
13. Richard A. Spritz, Molecular genetics of oculotaneous albinism,
Human Molecular Genetics, 1994, 3, pp. 1469-1475.
14. Giuseppe Prota, Melanins and Melanogenesis, Academic Press Inc
(London) Ltd, 1992, pp. 64.
15. Giuseppe Prota, Melanins and Melanogenesis, Academic Press Inc
(London) Ltd, 1992, pp. 68.
16. Giuseppe Prota, Melanins and Melanogenesis, Academic Press Inc
(London) Ltd, 1992, pp. 69.
17. http://www.geocities.com/arbadistrict8/CoatColorBiochemistry.doc
18. Johnathan L Rees, The Melanocortin 1 Receptor (MC1R): More Then
Just Red Hair. Pigment Cell Res. 13: pp. 135-140(2000)
19.
http://www.informatics.jax.org/searches/allele_report.cgi?markerID=MGI:99456
20. Nicholis I. Mundy, Nichola S. Badcock, Tom Hart, Kim Scribner,
Kirstin Janssen, and Nicola J. Nadeau, Conserved Genetic Basis of
a Quantitative Plumage Trait Involved in Mare Choice, Science, 203, pp.
1870-1873(2004)
21. Richard O. Prum and Scott Williamson, Reaction-diffusion models of
within-feather pigmentation patterning, Proc. R. Soc. Lond. B (2002)
269, pp. 781-792.
22. Yina Kuang, Isreal Biran and David R. Walt, Simultaneously
Monitoring Gene Expression Kinetics and Genetic Noise in Single Cells
by Optical Well Arrays, Anal. Chem., 2004, 76, pp. 6282-6286.
23. Jonathan M. Raser and Erin K. O'Shea, Noise in Gene _Expression:
Origins, Consequences, and Control, Science, 23 September 2005, Vol;
309, page 2010-2013
24. Joseph W. Quinn, A Pigeon Breeder’s Notebook: An Introduction to
Pigeon Science, 1971, pp. 76.
25. E. Haase, S. Ito, A. Sell, and K. Wakamatsu, Melanin Concentrations
in Feathers from Wild and Domestic Pigeons, Journal of Heredity, 1992,
pp. 67-67.
26. Geoffrey E. Hill and Kevin J. McGraw, Bird Coloration, Vol 1,
Harvard University Press, Cambridge, Massachusetts, 2006, pp. 245.
Copyright 2006 by Richard Cryberg.
#
# #
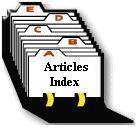
